FRET as a molecular ruler
FRET is the non-radiative transfer of energy by nearfield dipole-dipole coupling, which occurs after excitation of a fluorescent donor molecule with an spectrally and spatially overlapping acceptor molecule. The efficiency of the energy transfer is proportional to the inverse of the 6th power of the distance between the FRET pair and is scaled by the Foerster distance. The Foerster distance is a property of a particular FRET pair and itself depends on the spectral overlap of the emission of the
donor and the excitation of the acceptor and the orientation of the dipoles of the two fluorophores and at a spearation of r=R0 the energy transfer is 50%. The Foerster distance of the mTFP and Venus pair is 5.2nm and hence is most sensitive at a fluorophore separation of 5.2nm. Because each fluorophore has a radius of about 2nm plus the entropic spring which accumulates to nearly 5nm and therefore only efficiencies of <50% can be measured theoretically. How does the tension sensor module measure forces? In this sensor, the donor and acceptor fluorophores are separated by an elastic spider silk flagelliform, which acts as a molecular spring. A force across the spring tends to separate the fluorphores, which is detectable by a change in FRET. If the FRET cassette (later on called TSMod) is incorporated into a host molecules which is under tension, the force across the module will pull the two fluorophores apart with a distance proportional to the spring constant of the elastic spider silk domain. The increase in distance can then be measured as a decrease in the fluorescence intensity of the aceptor channel, indicative as a decrease in FRET. The intensity base FRET imaging, however, is prone to various artifacts such as crosstalk between the individual channel. Such a bleedthrough is unavoidable due to the nature of the overlapping emission and excitation spectra of the donor and acceptor fluorophor respectively.
Hence, the raw FRET image has to be corrected for such bleedthrough, which can be done by linear unmixing. Therefore, images have to be acquired at the same conditions and microscope settings from sample specimens that express either the donor molecule or the acceptor molecule alone. For example, no fluorescence should be recorded ideally after expression of the acceptor only upon excitation at the donor wavelength. This is never true. This is why FLIM is generally better accepted in the community, since the read-out, the change in fluorescence lifetime, is a physical property of the fluorophore and not of the imaging system.
FRET to study mechanosensation
The ability to sense mechanical forces is a ubiquitous property of all cells including neurons. Because all animals cells are viscoelastic, the stimulating force causes a deformation which in turn leads to a conformational change of a mechanosensitive ion channel. In living animals however, the source of the force is never coincident with the sink, but is transferred through viscoelastic material, e.g. from the skin to the nerve cell. The techniques available to study such a force transmission pathway in cells are limited and just emerging. Here present a simple yet powerful protocol to monitor the mechanical state of individual molecules with piconewton resolution in living animals.
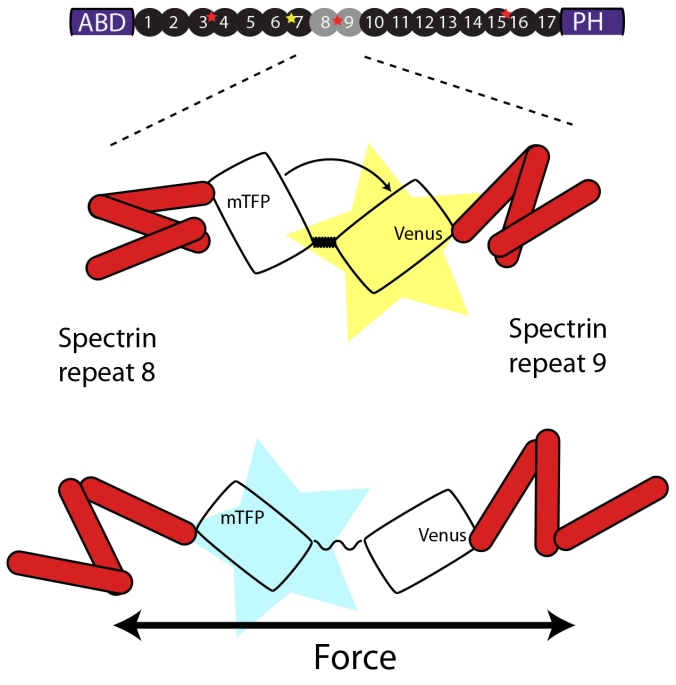
A FRET cassette consisting of a mTFP and Venus fluorophore connected by spider silk protein, is inserted into the middle of beta-spectrin – a region previously known to be subjected to force-induced conformational changes. The cassette can reproducible report forces on b-sspectrin in living animals.
The method is based on a recently published FRET sensor (Grashoff, Nature, 2010) that can be incorporated in any molecules of interest and imaging can be either performed on a standard confocal microscopy or epifluorescence microscope available to every lab or imaging facility. Quantitative FRET imaging is mot commonly performed on sophisticated instrumentation, such as fluorescence-lifetime-microscopy, which involved time correlated photon counting using detectors capable of counting single photons and a pulsed femtosecond laser – equipment not readily available to every researcher. To enable quantitative FRET microsocpy based on sensitized emission, we suggest construction of pre-calibrated ‘FRET-rulers’, in which the elastic spring is replaced by short 5 residue linker or by a large separation domain, whose FRET efficiencies have previously been measured with FLIM. Additionally, we provide a protocol to calibrate the photodetectors to adjust the gain setting and eventually correct the final FRET signal according to the collection efficiency of the detector. The calibration also enables us to estimate the error of each pixel and exclude values from the final quantification based on its uncertainty. We also implement an automatic neurite tracing algorithm, which tracks the boundary of the axon based on its Gaussian intensity distribution.